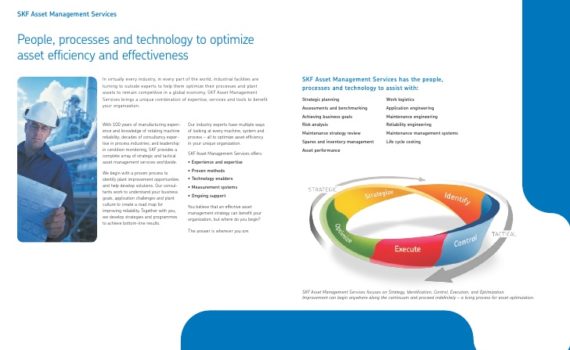
Spare parts management
Category : Technical Articles
Better management of spare parts inventories can unlock a hidden source of profit, explains Dr. Liang Dong, Service & Solutions Offer Manager at SKF.
Machines need spare parts. Any business operating significant quantities of machinery will carry of a stock of replacement components. Those parts fulfill a variety of purposes. They are there to replace items that wear out during normal operations, such as bearings, seals and filters and they need to be ready for planned upgrades and overhaul activities. They act as insurance, allowing maintenance teams to fix breakdowns faster than the lead times required to secure replacements from an external supplier.
Ensuring the organisation has the right number of the right spare parts in its inventories can be a continual source of tension, however. Maintenance and operations teams want to maximise availability, to reduce the risk of unplanned downtime and lost production due to missing parts. Finance staff want to minimise the valuable capital tied up on the shelves, and they worry about the obsolescence costs associated with parts bought for equipment no longer in use.
Both sides are right. Research by SKF has shown that optimising spare partsmanagement can reduce inventory budgets and holding costs by 15 to 20 percent, while simultaneously cutting stock-outs (and the resulting lost production) by 30 to 50 percent.
But how does a company achieve those optimal inventories? The trick lies in a better understanding of the organisation’s assets, of the spare parts they require, and of the nature of demand for those parts.
Some parts are all the same
Most organisations maintain a register of their assets, together with a database of the spare parts required in the support of those assets. Often, however, such partsdatabases have been developed organically over time, leading to inconsistency and duplication. Parts for two versions of the same asset may be listed in different ways on the database, for example, and simple standard parts like belts or switches may appear on the database under different names. This duplication matters because it reduces inventory efficiency. A company may order additional versions of the same part, since the database doesn’t show that they already have an appropriate item in their inventory.
Eliminating this waste requires database standardisation. The best companies use a common architecture for assets in their database, and standard maintenance bill-of-materials (BOM) structures and catalogue descriptions for the spare parts associated with those assets. In our experience, it is common for companies to be able to reduce the number of items in their spare parts inventories by 10 to 15 percent, just by eliminating duplicate or obsolete items.
Understanding demand
Once it knows which parts its assets require, a company needs to decide how many of each part it should keep in its inventory. Getting that right calls for an understanding of the way demand patterns vary, according to the nature of the part, and of the criticality of the asset to which it belongs.
Demand for spare parts falls into three basic categories: consumable spares, operational spares and insurance spares, and the best forecasting strategies for each are very different.
Consumable spares are items such as filters and lubricants. Typically, they are lower cost objects used in quite large numbers. When companies look at their historical consumption of spares like these, they will see a record of relatively level demand over time. Setting the optimal inventory level for these parts is a matter of establishing the quantity required to meet the overall average level of demand, plus an appropriate safety stock. That figure can then be refined to account for seasonal variations in demand as production patterns change, and updated over the longer term as the company makes alterations to its asset base.
Operational spares are items like fans and motors, for which demand is intermittent and unpredictable. Setting the right inventory level for these parts requires a more sophisticated statistical approach. By analysing historical usage, companies can gain an understanding both of their average consumption of these parts and its variability. They can also build a picture of the mean, minimum and maximum lifetimes for parts in service.
In practice, the lifetime of operational parts usually follows a “bathtub” curve. Some parts fail early, typically as a result of manufacturing or installation defects. After some time, these early failures tail off and the failure rate falls to a low level of random events. Finally, as parts get older, they begin to wear out, and the failure rate rises again. By applying different statistical distributions to each of these three causes of failure, companies can build a picture of the probability of failure of a particular type of part at any point in its life.
They can then establish an appropriate service level for the part in question and set their inventory targets to meet that level, given the probability of failure and lead time required to obtain extra parts from the original supplier. The right service level will depend on the price of the spare and the criticality of the asset. A 10 percent probability of a stock-out for a motor that runs one of six ventilators in a building may not create a significant problem, for example. An identical motor used to run a vital production machine will require a much higher level of availability, however.
The final category, insurance spares, requires a very different approach. These are typically high value parts for critical assets with very long supply lead times. Analysis of historical consumption is unhelpful in setting inventory levels for such parts, since the company may have consumed few, or none, of the part in the past. Likewise, it is very difficult to make meaningful estimates of the risk of the part failing in service in the future. One powerful way to make decisions about these kinds of part is to use a return-on-investment (ROI) approach to prioritise spend.
To do this, the company calculates the likely financial impact of the part failing with no immediate replacement available, by multiplying the cost of lost production by the lead time required to obtain a new part, and dividing this figure by the cost of keeping a replacement part in inventory. These ROI calculations allow the company to prioritise its expenditure on insurance parts. It may, for example, decide to stock only parts above a certain a level of ROI, or it may allocate a fixed budget to insurance parts, starting with the highest ROI items and working down the list until the budget is exhausted.
A basis for continuous improvement
Getting spare parts inventory levels right usually delivers significant improvements in both cost and asset availability. For the best companies, that is only the start, however. They monitor part consumption on an on-going basis to identify exceptions that may indicate an underlying issue with assets or operating practices. If a particular machine starts to experience an unexpectedly high number of early-life bearing failures, that might suggest an issue with improper assembly or lubrication. Spotting these trends in parts consumption allows the company to launch root-cause analysis efforts to understand and rectify the source of the problem.
A similar approach can be used to prioritise reliability improvement efforts. If a particular machine or category of parts is contributing disproportionately to consumable or operational part costs, the company may choose to launch a kaizen, or improvement, effort to tackle that machine’s performance and reliability. Alternatively it may consider the installation of condition monitoring technology to aid the early identification of problems, or could choose to invest in alternative technologies that offer greater reliability.
These efforts lead to a virtuous circle. As machine reliability improves and spares consumption falls, companies can alter their inventory parameters accordingly, freeing up further capital and reducing carrying costs. And as overall usage patterns change, it becomes easier to spot the remaining outliers, helping to focus future improvement efforts.
Help from the experts
While the payback from better management of spare parts is usually large, the journey to world-class performance can be daunting. To help companies on their way, SKF has developed the Spares Inventory Management and Optimization (SIMO) service. Built on more than 100 years of deep experience in rotating equipment performance and asset management, bearing lifetime management and supply chain management, SIMO provides a structured process designed to guide and support companies through every step of that journey.
SIMO is a four phase process, encompassing both the demand and supply aspects of spare parts management. It comprises spares identification and cataloguing, consumption forecasting, inventory rationalisation and on-going inventory optimisation.
As well as a rigorous, proven process, SKF brings important technical knowhow to the SIMO service. Clients can make use of standard spares templates for many common asset types, for example, aiding the development of a robust parts database. SKF has also built up a detailed benchmarking database of equipment performance across a range of industries, helping companies to pick the right forecasting models and to identify areas where their own equipment reliability or typical part service life falls outside of industry norms.
SIMO also integrates seamlessly with other SKF services designed to help companies minimize the total cost of ownership of their assets. Rather than buying individual bearings, for example, customers can use SKF’s “Bearings for Life” concept, in which they agree a price based on the total lifetime of an asset. SKF then supplies replacement components as required during that time, with SIMO calculations helping to determine appropriate on-site inventories. Likewise, SKF Logistics Services can use the same data to manage customer spares inventories on a consignment basis. And for every customer, SKF’s global distribution network ensures that huge number of spares are always available from stock or with short lead times, reducing the number of partsthey need to hold on site.
Every journey must start with the right first steps. To help companies to estimate the potential value of a shift to world-class practices, SKF has also developed CNA-SIMO (Customer Needs Analysis – SIMO), a simple but detailed needs analysis tool, allowing them to understand the current maturity of their spare parts management processes, and the principal opportunities for improvement.